QM Military: Quantum Encryption
<< Sass Pordoi, Dolomitis, Italy, JUL-27-2024 >> << Dallas Texas, MAY-20-2025 Re Factored for clarity >> TDM NOTE: This is a re factoring of this Article. We found the first version, like the subject matter, confusing. What is Cryptography ?? Before diving into the complex QM aspects of cryptography, lets first look at cryptography in general. Cryptography is the practice and study of techniques used to secure information and communications, ensuring that data is accessible only to those for whom it is intended. It involves methods of encoding (encryption) and decoding (decryption) information to protect it from unauthorized access, tampering, or theft. Cryptography plays a critical role in safeguarding digital communications, enabling secure transactions, protecting personal privacy, and maintaining the integrity of data across various platforms, such as emails, websites, and financial systems. It forms the foundation of modern cybersecurity. Encryption and Key Exchange Encryption is a method of securing information by transforming it into an unreadable format, called ciphertext, which can only be deciphered back into its original form, or plaintext, with the correct key. This process protects data from unauthorized access and is fundamental to modern digital communication. There are two primary types of encryption: symmetric and asymmetric. Symmetric encryption uses the same key for both encrypting and decrypting data, while asymmetric encryption uses a pair of keys—a public key for encryption and a private key for decryption. Symmetric encryption is fast and efficient, making it ideal for encrypting large amounts of data. However, it suffers from a critical limitation: both the sender and the receiver must have access to the same secret key, which means the key must somehow be shared securely beforehand. Common symmetric algorithms include AES (Advanced Encryption Standard) and DES (Data Encryption Standard). For example, if Alice wants to send Bob a secure message using AES, they must both have the same AES key, which they must agree upon privately. Asymmetric encryption, also known as public-key encryption, addresses the key distribution problem by using key pairs. A message encrypted with someone’s public key can only be decrypted with their private key. This makes it useful for secure communication over open networks. The most well-known asymmetric algorithm is RSA (Rivest-Shamir-Adleman). For instance, if Alice wants to send Bob a secure message, she can encrypt it using Bob's public key, ensuring that only Bob—who has the matching private key—can decrypt it. Key exchange protocols are cryptographic methods that allow two parties to securely share a secret key over a public channel. One of the most famous key exchange methods is the Diffie-Hellman key exchange, which enables two parties to jointly create a shared secret key even though they have never met and are communicating over an insecure channel. This shared key can then be used for symmetric encryption. While the exchanged key itself is not transmitted, the mathematical basis of the protocol allows both parties to compute it independently. A practical example of key exchange in action is the TLS (Transport Layer Security) protocol, which secures HTTPS web traffic. When you visit a secure website, your browser and the server use a combination of asymmetric encryption (e.g., RSA or elliptic curve cryptography) to perform a key exchange and agree on a symmetric session key. This session key is then used to encrypt the actual data transmitted between your browser and the server, providing both confidentiality and performance. As quantum computing advances, traditional encryption methods face potential threats, particularly those based on integer factorization and discrete logarithms (like RSA and Diffie-Hellman). This has spurred the development of post-quantum cryptography—new encryption and key exchange methods believed to be secure even against quantum attacks. Examples include lattice-based cryptography and code-based schemes. The future of encryption will depend on these emerging technologies to ensure data security in the quantum era. Now that we have looked at cryptograhy in general,lets see how QM can improve it. Here's how key quantum principles we looked at in earlier articles are used in encryption. Superposition Superposition allows quantum particles (like photons) to exist in multiple states simultaneously. In QKD, information is encoded in quantum states—often the polarization of photons—that are in a superposition of 0 and 1. Example (BB84 protocol): Alice sends qubits to Bob in randomly chosen bases (e.g., rectilinear or diagonal). Since each qubit exists in a superposition, an eavesdropper (Eve) cannot measure them without disturbing the state. Security implication: If Eve tries to measure the qubits, the act of measurement collapses the superposition, introducing detectable errors. Entanglement Entanglement is a phenomenon where two or more quantum particles become linked so that the state of one immediately affects the state of the other, regardless of the distance between them. Example (E91 or Ekert protocol): Alice and Bob share pairs of entangled photons. Measuring one instantly determines the outcome of the other. Security implication: Any attempt by an eavesdropper to intercept or measure one of the entangled particles disturbs the entanglement, changing the correlation statistics. This alerts Alice and Bob to the presence of an intruder. No-Cloning Theorem OK, We didnt cover this phenomenon in the previous articles. We were trying to keep it light. Anyhow, This quantum principle states that it is impossible to create an exact copy of an unknown quantum state. Security implication: Unlike classical data, quantum states cannot be copied and retransmitted without detection. This prevents an eavesdropper from intercepting and duplicating quantum keys without introducing observable anomalies. Measurement Disturbance As stated in earlier sections, sampling in things in QM actually forces the particle out of superposition in to one of its many a probablistic basis states.In quantum mechanics, measuring a quantum state generally disturbs it. This principle underpins the security of quantum communication. A QKD Example In QKD protocols, if an eavesdropper tries to measure the quantum bits during transmission, their actions change the quantum states. These disturbances are detectable by checking for errors in the shared key. Here is an example of QKD with Protocol BB84. Alice prepares a series of qubits in random states (using superposition). She sends them to Bob over a quantum channel. Bob measures them using random bases. Later, they compare notes (over a classical channel) to determine which measurements were made in the same basis. If no eavesdropping occurred, they should share identical key bits. Any tampering introduces detectable errors due to the above principles. So this is Key (no pun intended !), quantum encryption doesn't encrypt messages directly—it secures the key exchange, making it theoretically immune to any computational attack, including those by quantum computers. This makes QKD a cornerstone of quantum-safe communication. So can Quantum Encryption be Cracked ?? Quantum encryption, particularly Quantum Key Distribution (QKD), is theoretically secure based on the principles of quantum mechanics. The security of QKD protocols, such as BB84 and the Ekert protocol, relies on fundamental laws like the no-cloning theorem and quantum measurement disturbance. In these protocols, the key feature is that any eavesdropping on the quantum channel will disturb the quantum states, alerting the communicating parties (usually referred to as Alice and Bob) to the presence of an intruder. Therefore, in theory, quantum encryption cannot be cracked by conventional means, as attempts to intercept the quantum communication will leave detectable traces. Theoretical Security and Real World Implementation Vulnerabilities The no-cloning theorem ensures that quantum states cannot be copied or perfectly duplicated. This makes it impossible for an eavesdropper (Eve) to intercept and copy the quantum key without being detected, as the very act of measuring or tampering with the quantum states would alter them. Additionally, quantum key distribution relies on entanglement or superposition to encode the key, making any eavesdropping detectable by measuring error rates in the transmitted data. If Alice and Bob detect unusually high error rates during their verification process, they will know that the communication has been compromised and discard the key. While the theoretical foundation of quantum encryption is robust, practical implementations of QKD systems can be vulnerable to certain attacks. One of the main challenges comes from the hardware and device vulnerabilities in real-world quantum systems. For instance, imperfections in single-photon detectors or laser sources can open loopholes that an attacker could exploit. In 2010, a team demonstrated a "quantum hacking" method by targeting imperfections in QKD devices' detectors, allowing them to eavesdrop on the communication without being detected. It should be noted that these vulnerabilities aren't due to flaws in the underlying quantum principles, but rather in the physical devices used to implement quantum protocols. Manufacturers are working on improving the resilience of QKD systems against such attacks, but the possibility of side-channel attacks remains an issue. These attacks exploit information leaking from the physical operation of the system, rather than breaking the quantum cryptography itself. One problem is Side-Channel Attacks. A side-channel attack targets weaknesses in the quantum system's components rather than the protocol itself. For example, an attacker could exploit imperfections in photon detectors or manipulate timing information to gain insights into the key. In some cases, hackers could trick the QKD system into thinking it is operating securely, when in fact, information is being leaked. Side-channel attacks are common in classical cryptography as well, where attackers might exploit things like power consumption or electromagnetic radiation to gather clues about the encryption process. One of the most well-known side-channel attacks on QKD was the blinding attack, in which eavesdroppers manipulate the photodetectors in QKD systems to force them into classical behavior, bypassing the quantum measurement disturbance that would normally trigger an alert. These practical vulnerabilities show that while the quantum principles are secure, the implementation can be an Achilles' heel if not carefully managed. Another implemetation vulnerability is Distance and Infrastructure Limitations QKD systems often face distance limitations in practical applications. In fiber-optic cables, for instance, quantum signals degrade over long distances, leading to potential data loss or security vulnerabilities. Quantum repeaters, which would extend the distance over which quantum information can be transmitted securely, are still in development. This distance problem has led to alternative approaches, such as satellite-based QKD, which bypasses the need for ground-based infrastructure. China’s Micius satellite has successfully demonstrated long-distance quantum communication using satellites, though this technology is costly and currently not scalable for widespread use. Another practical challenge is the need for trusted nodes in long-distance communication networks. These nodes act as intermediaries to help relay quantum keys between distant locations, but they also represent potential points of attack, as they must be secure and trusted. If a node is compromised, the security of the entire communication system could be at risk. In response to these practical vulnerabilities, researchers are developing quantum-resistant devices and countermeasures to protect against known attacks. Device manufacturers are working to close loopholes in photon detectors and improve the security of quantum key generation and transmission. New techniques, such as device-independent QKD, aim to make the security of the system independent of the quality of the devices, meaning that even if the devices are not perfect, the key exchange remains secure as long as the overall quantum principles are followed. So, is quantum encryption actually used today in the real worls ? Yes, quantum encryption—specifically quantum key distribution (QKD)—is being used in certain specialized areas today, although its deployment is still in the early stages compared to traditional encryption methods.Below we look at actual QKD protocols and their implementations. Current QKD Protocols and Their Uses Here’s a comparison of current quantum key distribution (QKD) protocols—including BB84, E91, and others—along with notes on real-world use. We also cite the Quantum Principle used. BB84 (Bennett-Brassard 1984) Type: Prepare-and-measure Mechanism: Uses polarized photons and random basis selection (rectilinear and diagonal) Quantum Principle: Superposition, measurement disturbance Used in practice? Yes – widely implemented in commercial QKD systems (e.g., ID Quantique, Toshiba) Deployment examples:SwissQuantum network (Switzerland) SEQURE project (EU) China’s Beijing–Shanghai quantum communication line E91 (Ekert 1991) Type: Entanglement-based Mechanism: Uses entangled photon pairs and Bell inequality tests to detect eavesdropping Quantum Principle: Entanglement, Bell's theorem Used in practice? Prototype – demonstrated in labs and pilot networks; harder to implement at scale than BB84 Deployment examples: Experimental setups like the European Space Agency, and the Chinese Micius satellite B92 (Bennett 1992) Type: Simplified version of BB84 Mechanism: Uses only two non-orthogonal quantum states instead of four Quantum Principle: Superposition, no-cloning Used in practice? Rarely – less secure and more vulnerable to intercept-resend type attacks SARG04 Type: BB84 variation for enhanced security in noisy channels Mechanism: Same states as BB84 but different sifting protocol Quantum Principle: Superposition, measurement disturbance Used in practice? Protoype – used in research settings, especially where photon number splitting (PNS) attacks are a concern DI-QKD (Device-Independent QKD) Type: Advanced entanglement-based protocol Mechanism: Relies on Bell inequality violations without trusting devices Quantum Principle: Entanglement, Bell nonlocality, no-cloning Used in practice? Not yet practical – high hardware demands; under active research Real-World Implementation Notes China has led large-scale deployments: Micius satellite: Entanglement-based QKD experiments with E91 Beijing–Shanghai quantum backbone: Commercial BB84-type systems Europe and Japan: Pilot projects and quantum networks using BB84 Private companies (e.g., ID Quantique, Toshiba, QuintessenceLabs) offer commercial QKD systems based on BB84 variants Summary BB84 is the most practical and widely deployed QKD protocol. E91 offers stronger theoretical security but is harder to implement. Newer protocols like DI-QKD are pushing the boundaries of quantum security but are not yet ready for deployment. The Problems of QKD Distance Limitations: Current QKD systems are generally limited to short distances due to signal degradation in fiber-optic cables. Quantum repeaters, which are necessary for long-distance quantum communication, are still in the development stage. Satellite-based QKD, like China’s Micius project, helps extend distances but is costly and complex. Cost and Infrastructure: The hardware required for quantum encryption, such as highly sensitive photon detectors and quantum communication channels, is expensive and still developing. This has limited its widespread commercial adoption. Integration with Classical Systems: Quantum encryption technology needs to be integrated with existing classical infrastructure, which poses challenges for widespread use, though hybrid systems are being developed. Future Growth of QKD Quantum encryption, especially QKD, is expected to become more widely used as technological advancements address the current limitations in cost, distance, and integration. Its importance is likely to grow with the eventual rise of quantum computers, which could break many of today's standard encryption methods. In summary, quantum encryption is already in use in high-security sectors and is being actively developed for broader commercial use, although its current application is limited to specialized contexts due to technical and infrastructure challenges. Quantum cryptography research is also exploring ways to combine classical cryptography with QKD to provide additional layers of protection. For instance, post-quantum cryptography, which is designed to withstand attacks from quantum computers, may be used alongside QKD to ensure security in both quantum and classical domains. Conclusion Cryptography fundamentally revolves around securing communication by encrypting information so only authorized parties can access it. Traditional methods include symmetric encryption, which uses the same key for both encryption and decryption, and asymmetric encryption, which uses a public-private key pair to resolve the problem of key distribution. Protocols like RSA and Diffie-Hellman are widely used for secure communication and key exchange, often forming the backbone of internet security via systems like TLS. However, these classical approaches are vulnerable to the capabilities of quantum computing, which can break key cryptographic algorithms by efficiently solving problems like integer factorization and discrete logarithms. Quantum cryptography—especially Quantum Key Distribution (QKD)—offers a fundamentally new approach based on quantum mechanics rather than computational difficulty. Protocols like BB84 and E91 leverage principles such as superposition, entanglement, and the no-cloning theorem to ensure that any eavesdropping attempts are detectable due to disturbances they introduce in the quantum states. While BB84 is already commercially implemented in networks like China’s quantum backbone and European pilot programs, E91 remains mostly in prototype form due to its reliance on entanglement and Bell test setups. Despite its theoretical robustness, real-world quantum encryption faces challenges such as hardware imperfections, side-channel attacks, and distance limitations. Ongoing research aims to mitigate these issues through improved hardware, satellite-based communication, and hybrid approaches that combine quantum and post-quantum cryptographic methods. One VERY worrying conclusion is that it appears China is the leader or a top contender in this VERY important field.
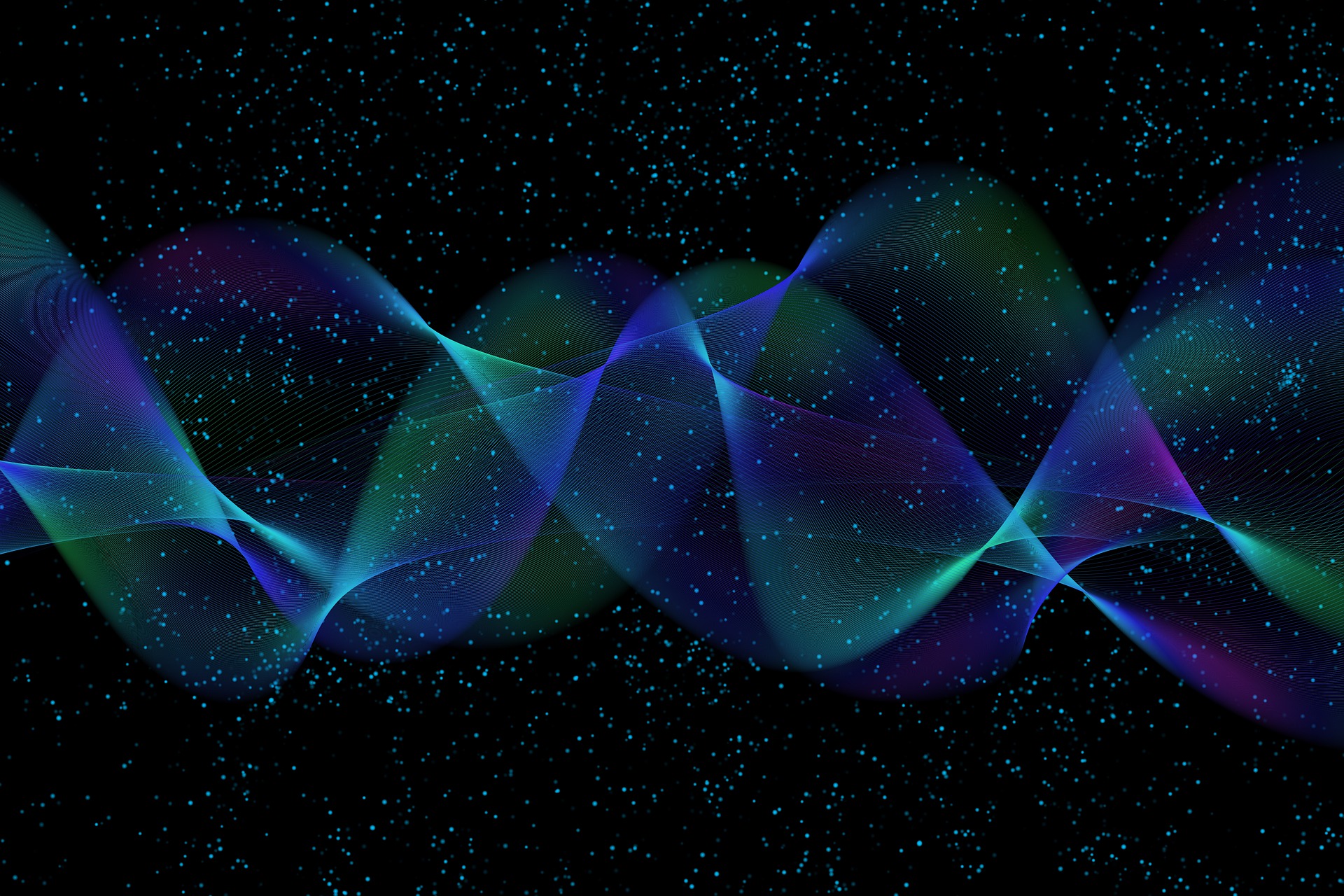